In the field of piping engineering, the integrity and reliability of materials under various operating conditions are of paramount importance. Among the various failure mechanisms that can compromise the performance of piping systems, creep failure stands out as a critical concern, particularly in high-temperature environments. This technical blog delves into the intricate details of creep failure, exploring its mechanisms, factors influencing its onset, methods of analysis, and strategies for mitigation. This comprehensive analysis is intended to provide a deep understanding of creep failure, empowering engineers to design more resilient piping systems.
What is Creep Failure?
Creep is a time-dependent deformation that occurs when a material is subjected to a constant load or stress over an extended period, particularly at elevated temperatures. Unlike other forms of mechanical failure, which are typically immediate and catastrophic, creep manifests gradually, often leading to the slow and progressive deformation of materials. This deformation can eventually result in failure, which is termed creep failure.
Mechanisms of Creep
Creep occurs in three distinct stages:
- Primary Creep (Stage I): This stage is characterized by a high initial rate of deformation, which gradually slows down as the material undergoes strain hardening. The material’s resistance to deformation increases, leading to a deceleration in the creep rate.
- Secondary Creep (Stage II): Also known as steady-state creep, this stage is marked by a constant creep rate. The material deformation progresses at a uniform rate, and this stage often constitutes the majority of the material’s creep life.
- Tertiary Creep (Stage III): In this final stage, the creep rate accelerates rapidly, leading to significant deformation and eventual failure. This stage is associated with the onset of microstructural changes, such as the formation of voids and cracks, which culminate in the material’s rupture.

Factors Influencing Creep Failure
Several factors influence the onset and progression of creep failure in piping systems:
- Temperature: Creep is highly temperature-dependent, with higher temperatures accelerating the creep process. For most metals, creep becomes significant at temperatures above approximately 0.4 times their melting point (in Kelvin).
- Stress: The applied stress is a critical factor in creep behavior. Higher stress levels can lead to a faster onset of creep and a reduction in the material’s creep life.
- Material Properties: Different materials exhibit varying resistance to creep. For example, materials with a higher melting point, such as nickel-based superalloys, tend to have better creep resistance compared to lower melting point materials like aluminum.
- Microstructure: The microstructure of the material, including grain size, phase distribution, and the presence of precipitates, plays a crucial role in determining its creep resistance. Fine-grained materials typically exhibit better creep resistance due to their ability to impede dislocation movement.
- Environment: Environmental factors, such as the presence of corrosive elements, can exacerbate creep by accelerating microstructural degradation and crack propagation.
Creep Failure in Piping Systems
In piping engineering, creep failure is a critical consideration, especially in systems that operate under high-temperature conditions, such as those found in power plants, refineries, and chemical processing facilities. Piping systems in these environments are often subjected to continuous high temperatures and stresses, making them susceptible to creep.
Creep in Different Piping Materials

- Carbon Steel: Carbon steel is commonly used in piping systems, but it has limited creep resistance at temperatures above 425°C. At elevated temperatures, carbon steel can undergo significant creep deformation, leading to thinning of the pipe walls and eventual rupture.
- Stainless Steel: Stainless steels, particularly austenitic grades, have better creep resistance than carbon steel. However, they are still susceptible to creep at temperatures exceeding 600°C, where the steady-state creep rate can become significant.
- Nickel-Based Alloys: Nickel-based superalloys, such as Inconel, are often used in high-temperature applications due to their excellent creep resistance. These materials maintain their mechanical properties even at temperatures exceeding 800°C, making them suitable for critical high-temperature piping systems.
- Creep-Resistant Steels: Specialized creep-resistant steels, such as P91 and P92, have been developed for use in high-temperature environments. These steels contain alloying elements like chromium, molybdenum, and vanadium, which enhance their creep resistance by stabilizing the microstructure at elevated temperatures.
Creep Testing and Analysis
Understanding the creep behavior of materials used in piping systems requires rigorous testing and analysis. Creep testing is conducted to determine the creep rate and creep life of materials under controlled conditions. The results of these tests are used to develop creep models that predict the long-term behavior of materials in service.
Creep Testing Methods
- Constant Load Creep Test: In this test, a specimen is subjected to a constant load at a constant temperature, and the resulting deformation is measured over time. The test provides data on the primary, secondary, and tertiary stages of creep.
- Stress Rupture Test: This test is similar to the constant load creep test but is conducted until the specimen ruptures. The time to rupture and the stress level are recorded, providing valuable information on the material’s creep rupture strength.
- Creep Fatigue Interaction Test: This test examines the combined effects of cyclic loading (fatigue) and creep. It is particularly important in applications where piping systems experience both high temperatures and cyclic stresses.
Creep Models

Several mathematical models have been developed to predict creep behavior:
- Norton-Bailey Law: This empirical model relates the creep strain rate to the applied stress and temperature, typically expressed as:

- Larson-Miller Parameter (LMP): The LMP is a widely used method for correlating creep rupture data. It is expressed as:

- Monkman-Grant Relationship: This empirical relationship links the time to rupture with the minimum creep rate, providing a quick estimate of creep life.
Case Studies of Creep Failure
Case Study 1: High-Temperature Steam Piping in a Power Plant
In a coal-fired power plant, high-temperature steam piping is subjected to continuous high-temperature operation, making it susceptible to creep failure. In one documented case, a section of steam piping made from carbon steel failed after 20 years of service. Metallurgical analysis revealed that the failure occurred due to creep-induced thinning of the pipe walls. The plant had operated at temperatures exceeding the recommended limits for carbon steel, leading to accelerated creep and eventual rupture. The failure highlighted the importance of using creep-resistant materials, such as P91 steel, in high-temperature applications.
Case Study 2: Creep in Petrochemical Processing Piping
In a petrochemical processing plant, stainless steel piping was used to transport high-temperature fluids. After several years of operation, leaks were detected in the piping system. Investigation revealed that the leaks were caused by creep-induced cracking at weld joints. The piping had been exposed to temperatures exceeding the creep threshold for stainless steel, leading to the initiation and propagation of creep cracks. The case underscored the need for regular inspection and monitoring of piping systems operating at high temperatures to detect early signs of creep damage.
Mitigation of Creep Failure
Mitigating creep failure in piping systems requires a combination of material selection, design considerations, and regular maintenance. The following strategies can help reduce the risk of creep failure:
- Material Selection: Choosing materials with high creep resistance is the first line of defense against creep failure. For high-temperature applications, materials like nickel-based alloys and creep-resistant steels should be considered.
- Design Considerations: Piping systems should be designed to minimize stress concentrations, which can accelerate creep. This includes optimizing pipe supports, reducing sharp bends, and avoiding abrupt changes in pipe diameter.
- Operating Conditions: Operating temperatures and pressures should be kept within the material’s creep limits. Monitoring and controlling these parameters can extend the life of the piping system.
- Regular Inspection: Non-destructive testing (NDT) techniques, such as ultrasonic testing and radiography, should be used to inspect piping systems for signs of creep damage, such as wall thinning and cracking.
- Life Extension Programs: For critical piping systems, life extension programs can be implemented, which involve detailed creep analysis, material testing, and potential replacement of at-risk components.
Advanced Techniques in Creep Analysis
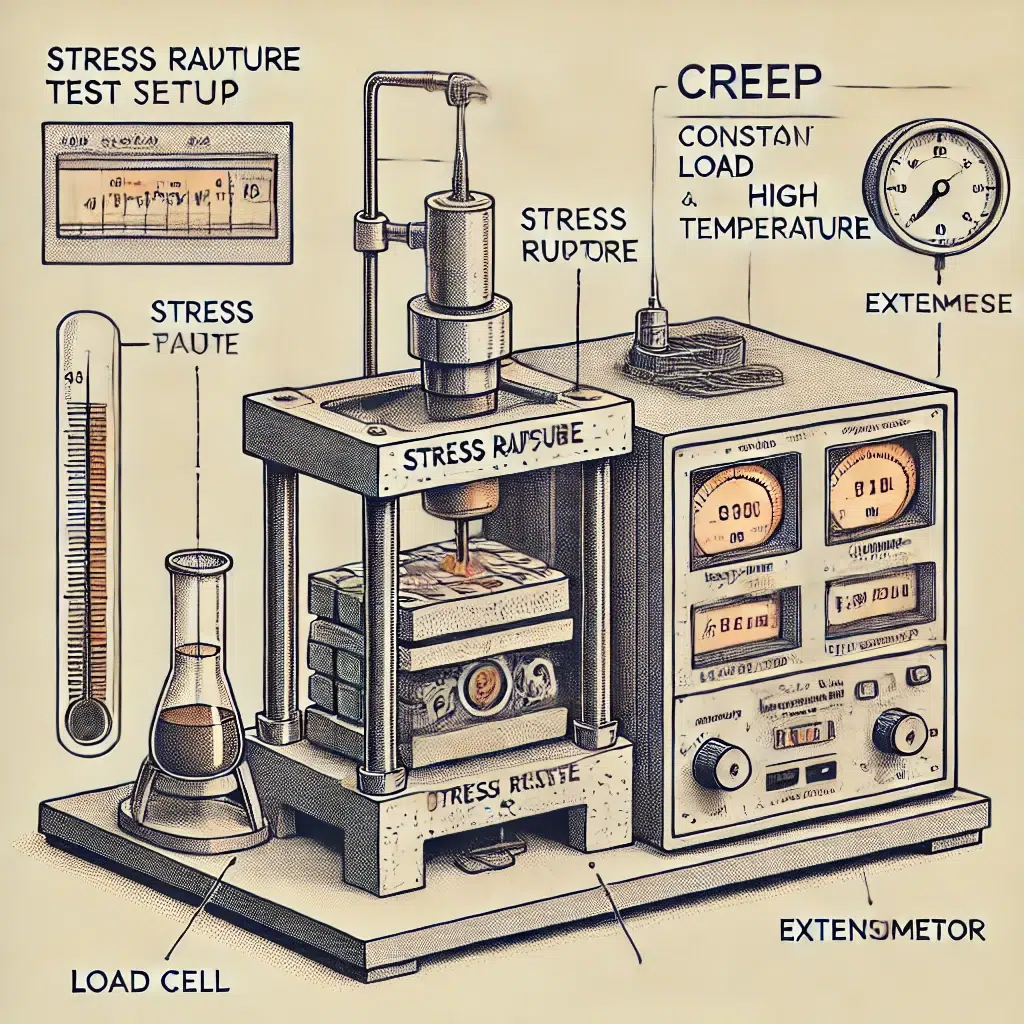
As the demand for higher efficiency and longer service life in industrial applications increases, advanced techniques in creep analysis have been developed to better understand and predict creep behavior.
Finite Element Analysis (FEA) for Creep
Finite Element Analysis (FEA) has become a powerful tool for simulating creep behavior in complex piping systems. By modeling the material properties, loading conditions, and environmental factors, FEA allows engineers to predict creep deformation and failure with high accuracy.
Creep Damage Modeling
Creep damage modeling involves the development of constitutive models that account for the microstructural evolution of materials under creep conditions. These models incorporate factors such as void nucleation, growth, and coalescence, providing a more detailed understanding of the creep process.
High-Temperature Material Development
Ongoing research in material science is focused on developing new materials with enhanced creep resistance. This includes the development of advanced alloys, such as ODS (Oxide Dispersion Strengthened) alloys, which exhibit exceptional creep strength due to the presence of fine oxide particles that impede dislocation movement.
Conclusion
Creep failure remains a critical challenge in piping engineering, particularly in high-temperature applications where materials are subjected to prolonged stress. Understanding the mechanisms, factors, and methods of analysis for creep is essential for designing resilient piping systems that can withstand the rigors of their operating environments.
By selecting appropriate materials, implementing robust design practices, and conducting regular inspections, engineers can mitigate the risk of creep failure and ensure the long-term reliability of piping systems. As technology advances, the development of new materials and analytical techniques will continue to enhance our ability to predict and prevent creep failure, safeguarding the infrastructure that supports modern industry.
In summary, creep failure is not just a material limitation but a complex interplay of factors that require careful consideration and management in the engineering of high-temperature piping systems.